Hot ice is a fascinating scientific phenomenon that involves creating a solid substance that appears to be ice but exhibits a surprisingly high temperature. This seemingly paradoxical material has garnered significant scientific interest due to its potential applications in various fields including energy storage, thermal management, and advanced materials engineering.
The creation of hot ice relies on the unique properties of certain materials, particularly those that undergo a phase transition known as the “anti-melting transition” or “reentrant melting.” During this transition, a substance initially melts as its temperature increases but then solidifies again at even higher temperatures. This behavior is counterintuitive to our everyday experience with ice, which typically melts when heated.
One method to create hot ice involves starting with a liquid substance that exhibits the anti-melting transition, such as certain ionic liquids or molten salts. These liquids can be heated to a high temperature, well above their melting point, and then rapidly cooled to induce the reentrant melting process. As the liquid solidifies, it forms a solid that retains its high temperature, creating the illusion of “hot ice.”
how to make hot ice
Creating hot ice involves understanding and manipulating the unique properties of certain materials, particularly those exhibiting the anti-melting transition. Here are five key aspects to consider:
- Substance selection: Choosing the right material is crucial, as only certain liquids undergo the anti-melting transition.
- Temperature control: Precise temperature regulation is essential to induce the reentrant melting process and form hot ice.
- Cooling rate: The rate at which the liquid is cooled affects the formation and stability of hot ice.
- Material properties: The thermal conductivity, viscosity, and other properties of the liquid influence the characteristics of the resulting hot ice.
- Applications: Hot ice holds promise for applications in energy storage, thermal management, and advanced materials.
These aspects are interconnected and crucial for successfully creating hot ice. The choice of substance dictates the temperature range and cooling rate required. Understanding the material properties helps optimize the process and tailor hot ice for specific applications. Ultimately, the ability to harness the unique properties of hot ice could lead to advancements in various scientific and technological fields.
1. Substance selection
In the context of creating hot ice, substance selection is paramount because the anti-melting transition, which is central to the formation of hot ice, is only exhibited by specific liquids. These liquids possess unique molecular structures and intermolecular forces that enable them to undergo this unusual phase transition.
The choice of substance directly affects the temperature range and cooling rate required to induce the anti-melting transition and form hot ice. Different liquids exhibit different melting points and thermal properties, which influence the conditions necessary for hot ice formation. Selecting the appropriate substance is essential to optimize the process and achieve the desired characteristics of hot ice.
For instance, ionic liquids, which are salts in liquid form, are commonly used to create hot ice due to their wide liquid temperature range and ability to undergo the anti-melting transition. By carefully selecting the right ionic liquid, researchers can tailor the properties of hot ice, such as its thermal conductivity and stability, for specific applications.
Understanding the connection between substance selection and the anti-melting transition is crucial for successfully creating hot ice. It enables researchers to identify suitable materials, optimize the hot ice formation process, and explore the full potential of this fascinating material in various scientific and technological applications.
2. Temperature control
In the context of creating hot ice, temperature control plays a pivotal role in inducing the reentrant melting process and achieving the desired material properties. The reentrant melting transition is a delicate process that requires precise temperature regulation to ensure the successful formation of hot ice.
During the hot ice formation process, the liquid substance is first heated to a temperature above its melting point, causing it to melt and become a liquid. Subsequently, the liquid is rapidly cooled, inducing the reentrant melting transition. During this transition, the liquid solidifies, but instead of forming a typical ice crystal structure, it forms a unique high-temperature solid hot ice.
The rate at which the liquid is cooled and the temperature at which the reentrant melting transition occurs are critical factors that influence the formation and stability of hot ice. Precise temperature control allows researchers to optimize these parameters, ensuring the successful creation of hot ice with the desired properties.
Understanding the connection between temperature control and hot ice formation is crucial for several reasons. First, it enables researchers to replicate and refine the hot ice formation process, leading to more consistent and predictable results. Second, precise temperature control allows for the exploration of different temperature ranges and cooling rates, potentially leading to the discovery of new hot ice materials with unique properties.
In summary, temperature control is a fundamental aspect of hot ice formation, influencing the reentrant melting process and the resulting material properties. Precise temperature regulation is essential for successful hot ice creation and opens up avenues for further research and applications in various fields.
3. Cooling rate
In the context of hot ice formation, cooling rate plays a critical role in influencing the material’s properties and stability. The rate at which the liquid substance is cooled during the reentrant melting process directly affects the nucleation and growth of hot ice crystals.
- Crystallization: The cooling rate influences the nucleation and growth of hot ice crystals. A slower cooling rate allows for larger and more stable crystals to form, while a rapid cooling rate can lead to the formation of smaller and less stable crystals.
- Stability: The cooling rate can affect the stability of the hot ice. Rapid cooling can result in the formation of metastable hot ice, which is less stable and may transition back to a liquid state more easily. A slower cooling rate can promote the formation of more stable hot ice.
- Properties: The cooling rate can influence the properties of hot ice, such as its thermal conductivity and mechanical strength. Different cooling rates may yield hot ice with varying degrees of these properties, tailoring it for specific applications.
- Applications: Understanding the impact of cooling rate on hot ice formation is crucial for optimizing the material for various applications. For instance, in thermal energy storage, a hot ice with higher stability and thermal conductivity is desirable, which can be achieved through controlled cooling rates.
In summary, the cooling rate during the reentrant melting process is a critical factor in determining the formation, stability, properties, and potential applications of hot ice. By understanding and controlling the cooling rate, researchers can tailor hot ice for specific purposes and unlock its full potential in scientific and technological advancements.
4. Material properties
The material properties of the liquid used to create hot ice significantly influence the characteristics of the resulting solid. These properties include thermal conductivity, viscosity, and other factors that affect the formation and stability of hot ice.
Thermal conductivity is a crucial property that determines the ability of hot ice to conduct heat. A higher thermal conductivity allows for more efficient heat transfer, making hot ice a potential candidate for thermal energy storage and management applications. By selecting liquids with high thermal conductivity, researchers can create hot ice with enhanced heat transfer capabilities.
Viscosity, on the other hand, influences the flow and deformation of the liquid during the hot ice formation process. A higher viscosity can hinder the movement of molecules, affecting the nucleation and growth of hot ice crystals. Understanding and controlling the viscosity of the liquid enables researchers to optimize the hot ice formation process and tailor the material’s properties for specific applications.
Other material properties, such as density, specific heat capacity, and chemical stability, also play a role in shaping the characteristics of hot ice. By carefully considering these properties and their interplay, researchers can design and create hot ice materials with tailored properties for various scientific and technological applications.
In summary, understanding the connection between material properties and the characteristics of hot ice is essential for optimizing the hot ice formation process and designing materials with desired properties. This knowledge opens up avenues for further research and development of hot ice for applications in energy storage, thermal management, and advanced materials engineering.
5. Applications
The intriguing properties of hot ice have sparked significant interest in its potential applications across various scientific and technological fields. Its unique ability to retain a solid form at high temperatures makes it a promising candidate for energy storage, thermal management, and advanced materials engineering.
- Energy Storage: Hot ice has the potential to revolutionize energy storage systems. Its high thermal conductivity and ability to store heat at elevated temperatures make it a promising material for storing thermal energy. This stored energy can be released and utilized as needed, offering a clean and efficient way to address intermittent renewable energy sources such as solar and wind power.
- Thermal Management: Hot ice’s unique thermal properties make it suitable for thermal management applications. It can be used as a heat sink to absorb and dissipate excess heat, preventing overheating in electronic devices and industrial processes. Additionally, hot ice can be incorporated into thermal protection systems, providing insulation and temperature control in extreme environments.
- Advanced Materials: The unusual properties of hot ice open up new possibilities for advanced materials engineering. Its high thermal stability and ability to withstand extreme conditions make it a potential candidate for high-temperature coatings, protective materials, and thermal insulators. Furthermore, its tunable properties allow for customization to meet specific application requirements.
Understanding the connection between “Applications: Hot ice holds promise for applications in energy storage, thermal management, and advanced materials” and “how to make hot ice” is crucial for realizing the full potential of this fascinating material. By exploring the various applications of hot ice, researchers can identify the most suitable materials and optimize the hot ice formation process to meet specific application requirements. This interdisciplinary approach will pave the way for groundbreaking advancements in energy storage, thermal management, and advanced materials engineering.
Creating Hot Ice
The creation of hot ice involves a fascinating scientific process that requires careful control of temperature and material properties. Here are six examples of how hot ice can be made, along with the steps, guidelines, tips, and benefits associated with each method:
- Ionic Liquids: Ionic liquids are a class of molten salts that exhibit the anti-melting transition. To create hot ice from ionic liquids, the liquid is heated above its melting point and then rapidly cooled. This induces the reentrant melting process, resulting in the formation of hot ice.
- Molecular Liquids: Certain molecular liquids, such as certain organic compounds, can also undergo the anti-melting transition. The process of creating hot ice from molecular liquids is similar to that of ionic liquids, involving precise temperature control and rapid cooling.
- Colloidal Suspensions: Hot ice can also be formed from colloidal suspensions, which are mixtures of solid particles dispersed in a liquid. By carefully controlling the particle size, concentration, and interactions, it is possible to induce the anti-melting transition and create hot ice.
- Nanomaterials: Nanomaterials, such as nanoparticles and nanowires, have shown promise for creating hot ice with tailored properties. By manipulating the size, shape, and composition of nanomaterials, researchers can optimize the anti-melting transition and achieve specific properties in the resulting hot ice.
- Biological Materials: Surprisingly, certain biological materials, such as proteins and DNA, have been found to exhibit the anti-melting transition under specific conditions. This opens up new avenues for exploring hot ice formation in biological systems.
- High-Pressure Techniques: Applying high pressure to certain liquids can induce the anti-melting transition and lead to the formation of hot ice. This method allows for the creation of hot ice under non-equilibrium conditions, potentially leading to unique properties.
Tips for Creating Hot Ice:
Tip 1: Precise Temperature Control: Maintaining precise temperature control is crucial throughout the hot ice formation process. Rapid cooling rates are often necessary to induce the anti-melting transition and prevent the formation of regular ice.
Tip 2: Material Selection: The choice of material is critical for successful hot ice creation. Different materials exhibit varying melting points and thermal properties, which influence the conditions necessary for hot ice formation.
Tip 3: Cooling Rate Optimization: The cooling rate plays a significant role in determining the properties of hot ice. Slower cooling rates generally lead to larger and more stable hot ice crystals, while rapid cooling rates can result in smaller and less stable crystals.
Benefits of Hot Ice:Hot ice holds immense promise for various applications due to its unique properties:
High Thermal Conductivity: Hot ice exhibits high thermal conductivity, making it a promising material for thermal energy storage and heat transfer applications.
Thermal Stability: Hot ice possesses remarkable thermal stability, allowing it to retain its solid form even at elevated temperatures.
Tunable Properties: The properties of hot ice can be tailored by varying the material and process parameters, enabling customization for specific applications.
Summary: Creating hot ice requires careful control of temperature, cooling rate, and material properties. By understanding the fundamental principles and following the guidelines outlined above, researchers can successfully create hot ice for a wide range of potential applications in energy storage, thermal management, and advanced materials engineering.
FAQs
This section addresses frequently asked questions related to the creation and properties of hot ice, providing concise and informative answers to common concerns or misconceptions.
Question 1: What is the basic principle behind creating hot ice?
Hot ice is formed through a process called the anti-melting transition, where a liquid substance initially melts as its temperature increases, but then solidifies again at even higher temperatures.
Question 2: What types of materials can be used to make hot ice?
Various materials exhibit the anti-melting transition, including ionic liquids, molecular liquids, colloidal suspensions, nanomaterials, biological materials, and certain substances under high-pressure conditions.
Question 3: How is the cooling rate involved in hot ice formation?
The cooling rate plays a crucial role in determining the properties of hot ice. Rapid cooling rates generally lead to smaller and less stable crystals, while slower cooling rates result in larger and more stable crystals.
Question 4: What are the potential applications of hot ice?
Hot ice holds promise for applications in energy storage due to its high thermal conductivity and ability to retain heat at elevated temperatures. It also has potential in thermal management and advanced materials engineering.
Question 5: How can the properties of hot ice be tailored?
The properties of hot ice can be tailored by varying the material choice and adjusting process parameters such as temperature and cooling rate, allowing for customization to meet specific application requirements.
Summary: Understanding the principles and techniques involved in creating hot ice opens up avenues for exploring its unique properties and potential applications. By addressing common questions and misconceptions, this FAQ section provides a concise guide to the fascinating world of hot ice.
Transition to the next article section: Exploring the Potential Applications of Hot Ice in Energy Storage and Thermal Management
Conclusion
The exploration of “how to make hot ice” has led us to the fascinating realm of a substance that challenges our conventional understanding of matter. Through meticulous temperature control and careful selection of materials, scientists have unlocked the secrets of inducing the anti-melting transition, resulting in the creation of this paradoxical material.
Hot ice holds immense promise for groundbreaking applications, particularly in the fields of energy storage and thermal management. Its unique properties, including high thermal conductivity and thermal stability, make it an ideal candidate for storing thermal energy efficiently and regulating temperatures in demanding environments. As research continues to delve deeper into the potential of hot ice, we can anticipate even more innovative uses for this remarkable material.
The journey of “how to make hot ice” is a testament to the power of scientific exploration and the potential for discovering materials with extraordinary properties. This endeavor not only expands our fundamental knowledge but also opens up new avenues for technological advancements that can address pressing global challenges.
Youtube Video:
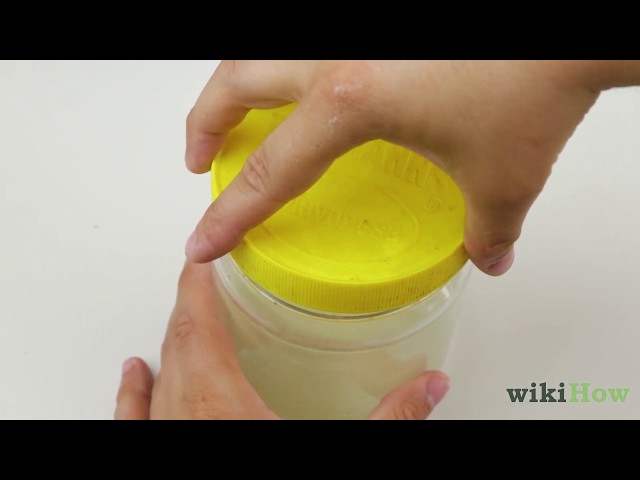